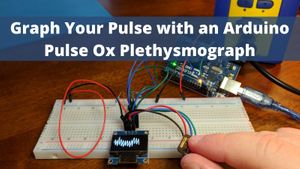
Graph Your Pulse with an Arduino Pulse Ox Plethysmograph
With the recent COVID-19 outbreak, I have noticed a new interested in biomedical engineering and electronics among the general population. In this tutorial, I’ll guide you through creating your own Arduino-based pulse plethysmograph.…